DNA sequencing was first developed more than five decades ago. Next-generation sequencing (NGS) methods have dominated for more than half that lifespan. First introduced in 2000, NGS technology incorporates massively parallel DNA sequencing techniques that power modern gene-based research.
This guide outlines how NGS sequencing works. We’ll review the evolution of NGS technology and methods, describe the five most popular types of NGS sequencing instruments, and suggest some best practices to support you in effectively incorporating NGS in your research or clinical lab.
- Evolution of NGS technology and methods
- The 5 most popular types of sequencing instruments
- Best practices to support NGS workflows
- Discover Sapio’s out-of-the-box NGS platform
Evolution of NGS methods
DNA sequencing has evolved through four generations of technology.
First-generation sequencing aka Sanger sequencing
In 1977, Frederick Sanger in the UK and Walter Gilbert in the U.S. independently developed first-generation sequencing, now known as Sanger sequencing. Sanger sequencing employs sequencing by synthesis (SBS). There are three steps to Sanger sequencing:
- Using chain termination polymerase chain reaction (PCR) to create and label a library of modified nucleotide fragments
- Separating oligonucleotides by size through capillary gel electrophoresis
- Using fluorescence to call the identity of each tagged oligonucleotide to determine the sequence of the input DNA
Today, scientists use Sanger sequencing to quickly and cost-effectively sequence low numbers of targets and samples. Scientists also use Sanger sequencing to confirm NGS results because of its accuracy and high data quality.
Second-generation sequencing, aka next-generation sequencing
Subsequent “generations” of DNA sequencing have been variants on next-generation sequencing (NGS). Massively parallel sequencing (NGS) utilizes SBS, but instead of separating sequencing reactions into lanes, capillaries, or tubes, sequencing occurs in parallel on glass or beads. The result is much higher throughput using smaller sample sizes, making sequencing more cost-effective than Sanger sequencing.
The most popular second-generation sequencers are Illumina sequencers, which account for 90% of the world’s sequencing data. Other second-generation sequencing instruments include BGI/MGI, GenapSys, Ion Torrent, and QIAGEN.
Third-generation sequencing, aka single-molecule sequencing
Second-generation sequencing has some drawbacks, mainly associated with using PCR to amplify the short reads of template DNA. Sequencing errors are common (hence the need to verify results with Sanger sequencing). In addition, scanning and wash cycles slow the process.
Third-generation sequencing approaches utilize single-molecule sequencing (SMS), sometimes called a single-template approach. The most well-known SMS method is single-molecule real-time (SMRT) sequencing, introduced by Pacific Biosciences (PacBio).
Fourth-generation sequencing, aka nanopore sequencing
Fourth-generation sequencing technology builds on SMS by incorporating an in situ sequencing method that uses second-generation sequencing chemistry to read nucleic acid composition directly in fixed cells and tissues. The most popular fourth-generation sequencing platform is nanopore sequencing, which identifies proteins, DNA, and RNA from the electric signal the molecules make when passed through nanoscale holes (nanopores) in a thin membrane. Oxford Nanopore manufactures the most well-known sequencers using nanopore technology.
The 5 most popular sequencers for NGS
These sequencing vendors, spanning second-generation to fourth-generation sequencing technologies, currently dominate the NGS landscape.
- Illumina sequencers account for 90% of the world’s sequencing data. Illumina pioneered SBS technology utilizing fluorescence to read clonally amplified DNA templates attached to a glass flowcell. Illumina offers a range of sequencers broadly categorized into benchtop sequencers (iSeq 100, MiniSeq, MiSeq, and NextSeq) and production-scale sequencers. (NextSeq and NovaSeq). The company discontinued its popular HiSeq platform in 2021; it will be supported through 2024.
- Ion Torrent sequencers were launched in 2011 as a direct competitor to Illumina. Ion Torrent sequencers employ second-generation SBS technology. Instead of using fluorescence to read sequences, Ion Torrent systems read nucleotide sequences using a semiconductor chip that measures changes in pH caused by the release of hydrogen ions during DNA polymerization. IonTorrent offers four different instruments. The GeneXus system is an entry-level system offering an economical option for low-sample throughput. IonGeneStudio S5 systems come in five variants and can generate 2M to 130M reads and 0.3 to 50Gb data in a single run taking at most 21.5 hours. The PGM Dx is a low-throughput diagnostic system.
- MGISeq instruments employ a form of second-generation sequencing called nanoball sequencing. Not to be confused with nanopore sequencing, SMS, MGI sequencers use rolling circle replication to amplify small fragments of genomic DNA into DNA nanoballs. The nanoballs are adsorbed onto a sequencing flow cell, and the sequencer reads the sequence using fluorescence. Aspects of the technology resemble Illumina technology, and in fact, MGI sequencers can accept sequencing libraries developed using Illumina protocols. The DNBSEQ-T7 has been reported to sequence up to 800,000 samples per year using an automated library preparation system that can operate for 24 hours without human intervention. MGI sequencing suffers from the same disadvantages as other short-read sequencing techniques and cannot resolve structural variants or distinguish highly homologous genomic regions. These instruments will likely gain more traction now that they are being marketed in the US and Europe.
- Oxford Nanopore markets its sequencers as portable and flexible, giving even the smallest labs access to NGS capabilities. Nanopore sequencing has made whole-genome sequencing faster and less expensive. It can also be used in conjunction with second-generation sequencing technology to identify the order of nucleotides in fixed cells and tissues. Oxford Nanopore released its first sequencer, the MinION, in 2014. It has since released the GridION Mk1 and the PromethION 24/48.
- PacBio HiFi sequencers use single-molecule real-time (SMRT) sequencing, a third-generation sequencing technology introduced in 2010. SMRT uses zero-mode waveguides (ZMWs) embedded on a chip. Each ZMW holds a single molecule of single-stranded DNA template, enabling scientists to visualize and monitor the activity of DNA polymerase in real time as synthesis occurs. SMRT can generate long reads at a low error rate and much faster than second-generation systems.
(Read more about popular NGS sequencers here)
Best practices to support NGS workflows
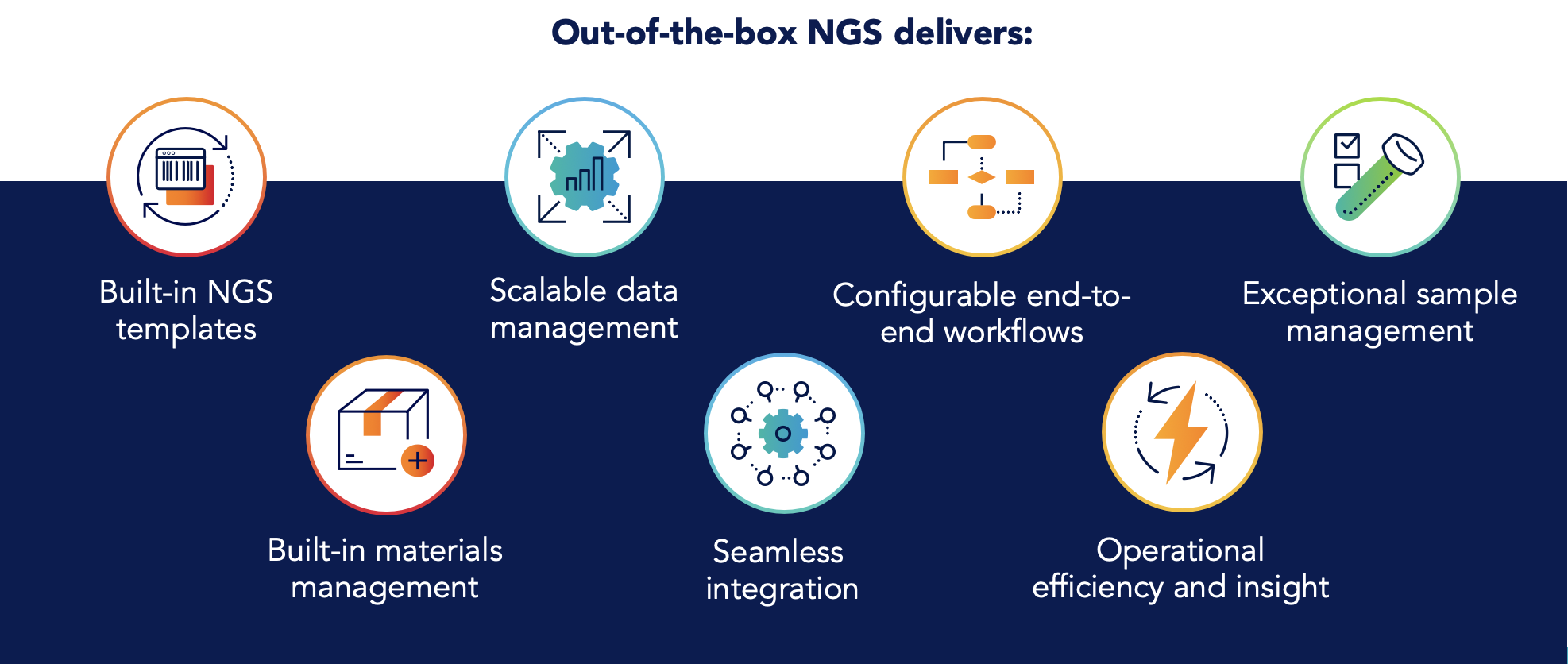
NGS workflows are complex and variable. Once a lab has selected a technology and NGS sequencing instruments to power its NGS, it must continually adapt and flex its NGS workflows across numerous steps and subtasks. Sequencing vendors provide sequencing kits that define standard protocols for scientists to follow. However, many labs still use folders, paper lab notebooks, Excel, and USBs to track samples, materials usage, and workflow steps. These methods simply can’t scale to manage the unprecedented amount of data generated by NGS.
The following best practices can help labs seeking to implement and support NGS.
Out-of-the-box preconfigured NGS templates
While NGS workflows inevitably change, basic tasks are predictable and required. All labs need ways to register and track materials; track and manage samples; communicate with instruments; and store and analyze data. Solutions that provide built-in templates out of the box ensure your lab can get up and running quickly.
End-to-end lifecycle sample management
NGS begins and ends with samples, from when they arrive in a lab with a sequencing request to their analysis. Whether your lab is conducting research or clinical NGS, samples often come from patients who may make life-or-death decisions based on the results. Any NGS informatics solution must deliver exceptional sample management capabilities. This includes tracking sample lineage and aliquots, automatically reprocess samples that fail quality control, and searching for samples to follow their progress through the workflow.
Built-in materials management
While many labs have separate systems for inventory management, these systems can’t keep pace with the speed of NGS. In addition, individual scientists often track their team’s private usage of kits and reagents. This prevents the larger organization from managing resources and keeping necessary supplies on-hand. A built-in system for NGS materials management ensures that organizations can effectively run workflows while minimizing waste and reducing costs.
Seamless integration
NGS labs use a range of different tools and instruments for sequencing, analysis, and more. It’s not feasible to expect manual processes to work in such an ecosystem. Informatics platforms must be able to automatically push and pull data on and off instruments, run metrics in real-time to ensure accuracy and efficiency, and even track maintenance schedules, calibration, and other priorities.
Operational efficiencies and insights
Visibility and transparency are essential in a fast-paced laboratory environment. As discussed above, automation can enable scientists to spend less time manually entering information and more time doing science. With dashboards and visualizations, scientists and lab managers can track work progress, flag key performance indicators, and collaborate effectively with colleagues in the same lab, down the hall, or at partner sites. Custom views based on scientific roles ensure scientists have access to all the information they need to stay informed and keep work moving productively.
Discover out-of-the-box NGS from Sapio
Read our comprehensive NGS Toolkit guide to learn more about how an out-of-the-box NGS solution can power your lab’s science. Or, if you’re ready to see how the system can help your lab now, request a free demo today.